The Evolution Toward OTN —
Just as oxygen and water are critical to human life, so is spectrum and fiber to the mobile operator. In her June 2017 article in ISE magazine, Heather Burnett Gold, President and CEO of the Fiber Broadband Association, outlined how critical fiber is to the mobile operator and how this is driving significant M&A activity in the industry; billions of dollars are spent each year to acquire these precious resources.* Unlike any other technology, fiber provides the bandwidth, low latency, and quality of service, that will be required to make 5G networks a reality. Let’s look a little deeper to understand why.
The Critical Building Block
While the initial LTE rollout has mostly completed, operators are now focused on 2 primary activities:
1. Adding capacity to their 4G network with LTE-Advanced and cell-site densification.
2. Preparing for 5G.
Both these megatrends are causing mobile operators to embrace the centralized radio access network (C-RAN) architecture. C-RAN is an architecture in which mobile baseband units (BBUs) are no longer placed at the bottom of a cell tower, but instead are placed in a centralized location and only remote radio heads (RRHs) are left at the tower. This reduces operator CapEx and OpEx while boosting capacity by enabling cell coordination amongst the now centralized BBUs. Evolving to the C-RAN architecture is also a critical first step towards introducing virtualization and cloud technologies into the radio access network (RAN).
Once the BBUs are centralized, a high capacity and low latency fronthaul network must be introduced. In 4G, the fronthaul network will transport the common public radio interface (CPRI) between the BBUs at the centralized location and the RRHs at the cell-sites. The CPRI specification provides for a range of transmission rates that are directly related to the carrier bandwidth and the number of antennas used per RRH. As operators evolved from 4G/LTE deployments based on 2×2 MIMO and 20 MHz carriers to 4×4 or 8×8 systems, the CPRI rate scaled from 2.5 Gbps to 9.8 Gbps. A fronthaul network servicing a single three sector cell-site would therefore need a capacity of roughly 30 Gbps.
5G will unleash a combination of wider carrier bandwidths and massive MIMO featuring hundreds of antennas per radio head, which in turn will drive a substantial increase in the RRH bandwidth to upwards of 100 Gbps per sector. The fronthaul network will now need a capacity of several hundred gigabits/second per cell-site. (See Figure 1.)
Figure 1. Mobile Network Evolution from 4G through 5G
High bandwidth is not the only requirement for fronthaul. In order for the mobile network to adhere to strict timing requirements set forth by 3GPP, the fronthaul network must also offer exceptionally low end-to-end latency, generally in the range of 50 µs-150 µs.
Lastly, fronthaul must also be multiservice, supporting both CPRI-based 4G equipment and Ethernet-based 5G equipment. Fiber is the only medium on which the high bandwidth, low latency, and multiservice fronthaul network that 4G and future 5G networks require can be built.
Fronthaul Options
With the importance of fiber established, the question becomes what to put over it. While directly connecting RRHs to BBUs with fiber is possible, transporting a single CPRI or Ethernet client over a fiber is a costly and fiber-inefficient way to deploy a fronthaul network. A simple cell site with 3 RRHs would need 6 fiber strands. Consequently, some method must be found to aggregate multiple CPRI and Ethernet clients onto a fiber in a cost-effective manner while meeting the bandwidth, low latency, and multiservice requirements.
Passive and active WDM-based fronthaul systems are one alternative. They reduce cost and increase efficiency by transponding each CPRI or Ethernet onto its own wavelength, and then wavelength multiplexing them onto the fiber. These systems offer a capacity of Nx10G, where N is the number of wavelengths supported (typically 16 or 44). Furthermore, these systems offer extremely low latency.
However, WDM systems do suffer from several disadvantages, particularly as we consider the evolution to 5G.
Not 5G ready — Conventional WDM fronthaul systems are limited to 10G wavelengths due to a lack of available higher-rate WDM modules that are also industrial-temperature rated. This is a fundamental disconnect with 5G which, at least for the foreseeable future, will require support for Nx25GE per radio head.
Limited scalability — Once the available wavelengths have been consumed, more fiber and more equipment must be deployed to handle the required capacity.
Higher power — WDM optical modules are higher power. This can lead to incompatibility with existing RRHs, as well as higher OpEx.
Complex inventory management — "Fixed" wavelength WDM optical modules are typically used. Because each wavelength must be unique for each CPRI client, the operator must maintain inventory for each of the WDM wavelengths in use. Furthermore, when a module fails, it is critical that it be replaced with one of the same wavelength. This can be problematic under any situation, let alone during outdoor tower climbs.
With 5G equipment moving away from CPRI towards Ethernet interfaces, Ethernet-based fronthaul is garnering substantial industry interest. While Ethernet is attractive, particularly where a mobile operator is using third-party transport, we’re likely several years away from standards and technologies being in place to enable its widespread adoption in the RAN. Furthermore, 4G-based mobile equipment with CPRI interfaces will still be deployed for many years to come. In order to avoid deploying multiple parallel fronthaul networks, mobile operators need a transport option that not only offers high bandwidth and low latency, but offers the multiservice capabilities to enable a converged 4G and 5G fronthaul network. Enter the ITU-T’s standardized optical transport network (OTN).
The Case for OTN
The evolution currently taking place in the mobile network to dense 10G CPRI interconnect, coupled with the need for Nx25G Ethernet, has many parallels with the evolution of today’s metro network. As the services in the metro network evolved from T1/E1 to today’s multiservice network supporting GE, 10GE, SONET/SDH, video, and FC/SAN, the transport layer evolved from 10G SONET/SDH to 100G OTN. (See Figure 2.)
Figure 2. Mobile Network Requirements Parallel the Metro Network Evolution
The ITU-T’s G.709 OTN standard enables a single transport layer that simultaneously supports multiple client services and rates coupled with carrier-grade OAM. OTN allows 100G or more of multiservice clients to be multiplexed into the same 100G container (OTU4) with sub-1 µs latency possible. Furthermore, as volume ramps, the ecosystem is continuing to drive cost out, allowing 100G OTN to provide substantial cost/bit savings relative to Nx10G WDM. As a result, 100G OTN has become the dominant transport layer used in service provider metro networks worldwide. The same characteristics that drive 100G transport in the metro is also what mobile networks require as they evolve to LTE-A and 5G.
Figure 3 shows how OTN transports 4G and 5G client services. Individual clients, whether they are 10GE, 25GE, or CPRI, are transparently mapped into their own low-order ODUk containers. These ODUk containers are then multiplexed into a high-order ODU4 container before being transmitted on the line using grey optics or WDM.
Figure 3. Transporting 4G and 5G Services over OTN
These low-order ODUks are switchable, protectable, and provide full, carrier-grade OAM, including trail trace and fault monitoring. These qualities ensure a highly reliable fronthaul network that can also dynamically allocate bandwidth as required.
With OTN as a building block, a converged 4G and 5G fronthaul network can now be built offering the high bandwidth, low latency, and multiservice characteristics, that are so critical. An example of such a fronthaul network is shown in Figure 4. In this example, multiple clients are aggregated into 100G OTN containers and optionally switched through the fronthaul network to the 4G BBU and 5G DU locations.
Figure 4. 100G OTN Enables a Converged 4G and 5G Fronthaul Network
Figure 5 summarizes the many requirements of a fronthaul network and how OTN compares against both alternatives. As can be seen, OTN comes out ahead in most categories, including the critical categories of bandwidth, latency, and multiservice support.
Figure 5. Comparing Fronthaul Transport Options
The combination of these advantages means that OTN represents the most cost-effective, fiber-efficient, and scalable fronthaul network alternative. The challenge has been a lack of OTN-based fronthaul solutions that meet the strict 3GPP and CPRI timing requirements. Microsemi’s recently announced DIGI-G4-based fronthaul solution has changed the equation. The solution enables a high bandwidth, low latency, and converged 4G/5G fronthaul network over Nx100G OTN. Carriers can now realize the benefits of an OTN-based fronthaul network without sacrificing network performance, scalability, or flexibility.
Endnote
*"Fiber to 5G: Accelerating the Connected Future" by Heather Burnett Gold. ISE magazine, June 2017. https://isemag.com/2017/06/fiber-to-5g/
Save
About the Author
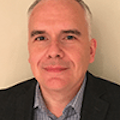